what’s the big deal?
Meteorites are just rocks, right? Then why are hundreds of scientists studying these ‘rocks’ all around the world? Sure, expanding human knowledge is cool, but we get more from studying meteorites than just that.
Some scientists study the primitive structures in chondrites, attempting to explain the chain of processes that took place as the first solid matter coalesced around our young sun. Why is this important? Meteorites are our only solid samples of pristine material from the formation of any planetary system. Understanding how chondrules and chondrites formed is integral if we’re ever to understand how solar systems ‘work’ — especially ones like our own that might harbor life.
Inclusions in carbonaceous chondrites like CAIs (calcium aluminum inclusions) and microscopic pre-solar grains can even tell us about what was going on in our solar system prior to the formation of the planetary nebula. That’s right, some meteorites even contain microscopic grains that must have formed directly within novae or supernovae. Mineral grains from long before our sun ever existed. In the past ~decade, advances in isotopic resolution have allowed us to study the nature of the stars that provided the raw material to make our sun and solar system!
Without meteorites, we would have to rely solely upon telescopic data (like the images below) in order to infer anything about the formation of our solar system, or the formation processes of planets in general. Meteorites are the solid remnants we have from a time when our solar system looked something like this:
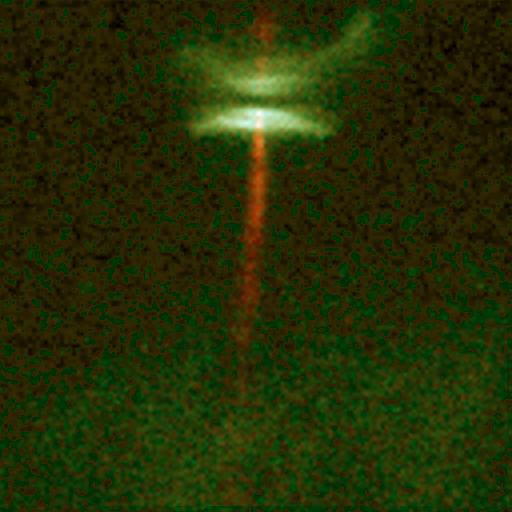
There’s plenty that we can glean from these images, but if we want to understand what happened in our solar system, with our planets, in relation to the Earth, etc., we’ve got to get a closer look at what’s going on in these images.
As of late 2014, our imaging ability has improved somewhat; here’s a new image of a sun-like star, HL Tau, taken by the Atacama Large Millimeter/submillimeter Array in Chile:
Meteorites allow us to take a much closer look at these kinds of environments. Those disks in the images above are ~100+ AU across. Much bigger than the orbit of Pluto (~30 – 49 AU). Distant stars and planetary systems like these can tell us a lot — but most meteorites are solid left-overs from back when the first rocks were forming directly out of our planetary nebulae, just like those. See here for more information on chondrites.
Other scientists focus on achondrites, hoping to understand how planetary evolution occurred in the period just after chondrites formed. Achondrites lack chondrules; they are pieces of planetesimals that have been, for the most part, destroyed by impacts. Some recent work even suggests that the first planetismals accreted only a few million years after chondrules formed (if not earlier!), which has big implications for our understanding of the early solar system.
This is an important idea; chondrites are comprised of ~millimeter-sized droplets of flash-heated rock that are cemented together with fine, dusty material. Older models suggested that accretion was a continuous process that resulted in the formation of larger bodies as smaller ones collided and stuck to each other. If large planets were forming around the same time as chondrules…it means that we have to rethink the relationships between chondrules, planets, and asteroids — and how and when each of those kinds of objects formed.
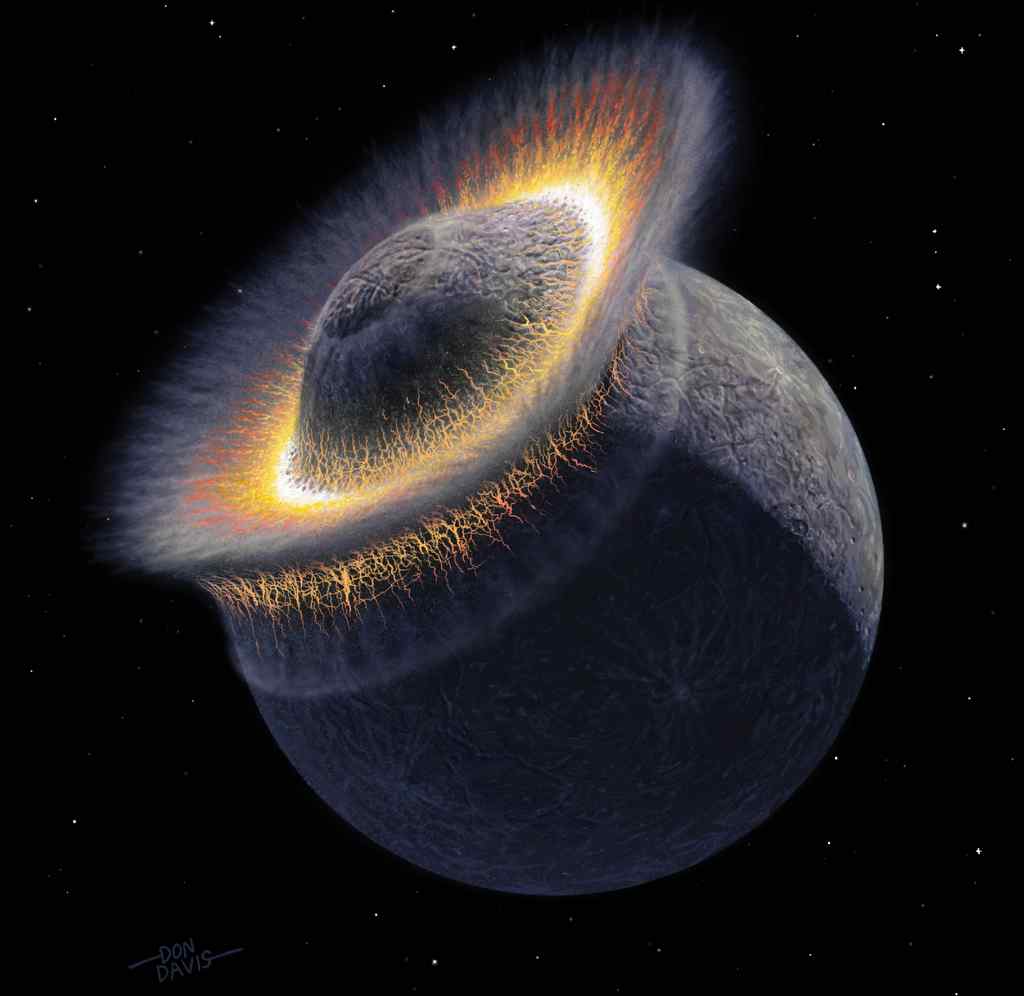
Achondrites also include rare meteorites from bodies like Mars and the Moon. These rocks have given us a much better understanding of both of these bodies, with applications to future human habitation.
While future Martian sample return missions are in their early development phases and will cost untold billions of dollars, it is important to remember that we already have hundreds of pounds of Mars here on Earth, ready for close examination. How do they get here? See here.
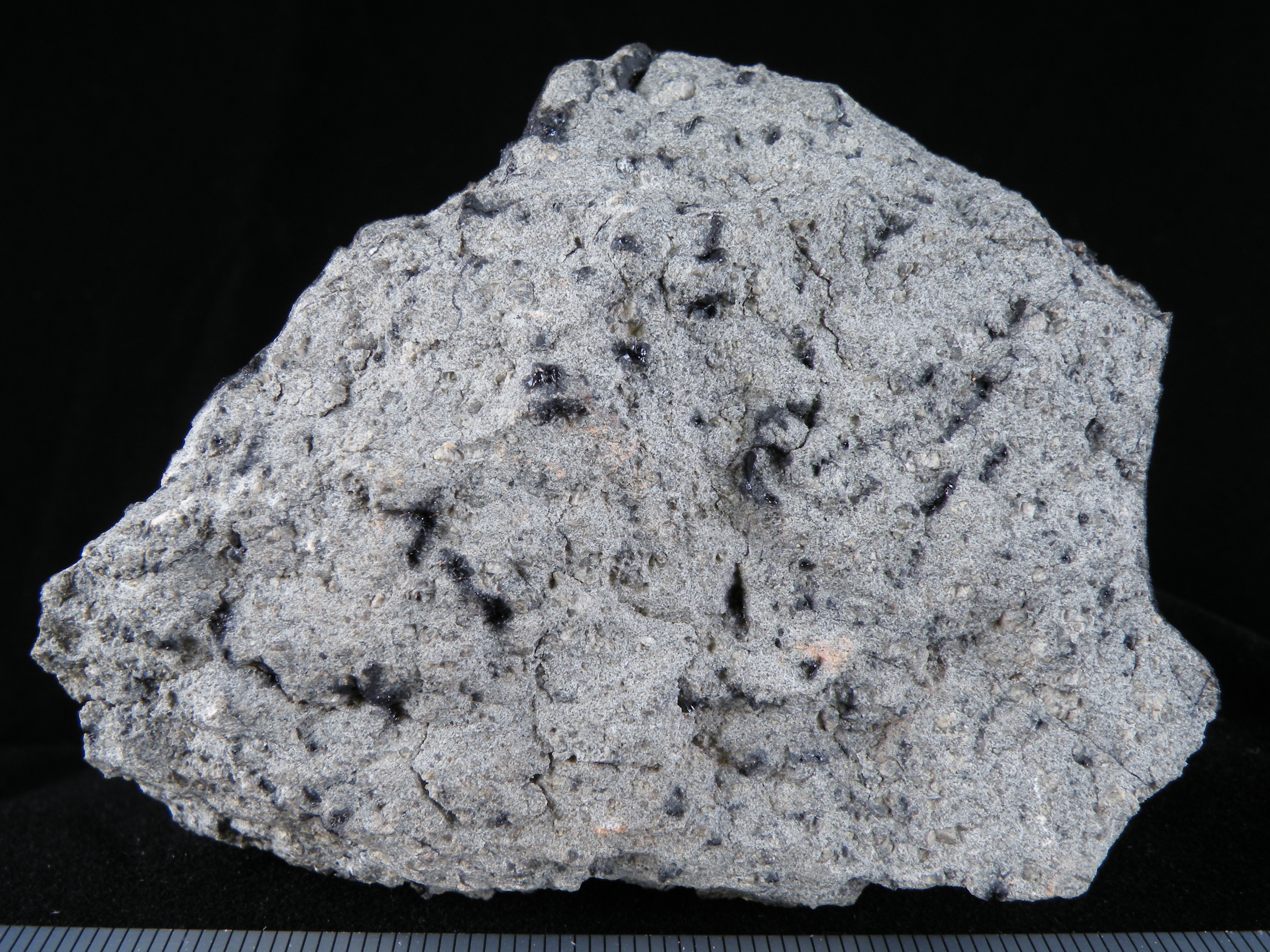
Lunar meteorites have given us a more detailed look at the Lunar surface than was possible with the limited sample collecting abilities of the Apollo missions, and the cost of these natural samples is negligible in comparison.
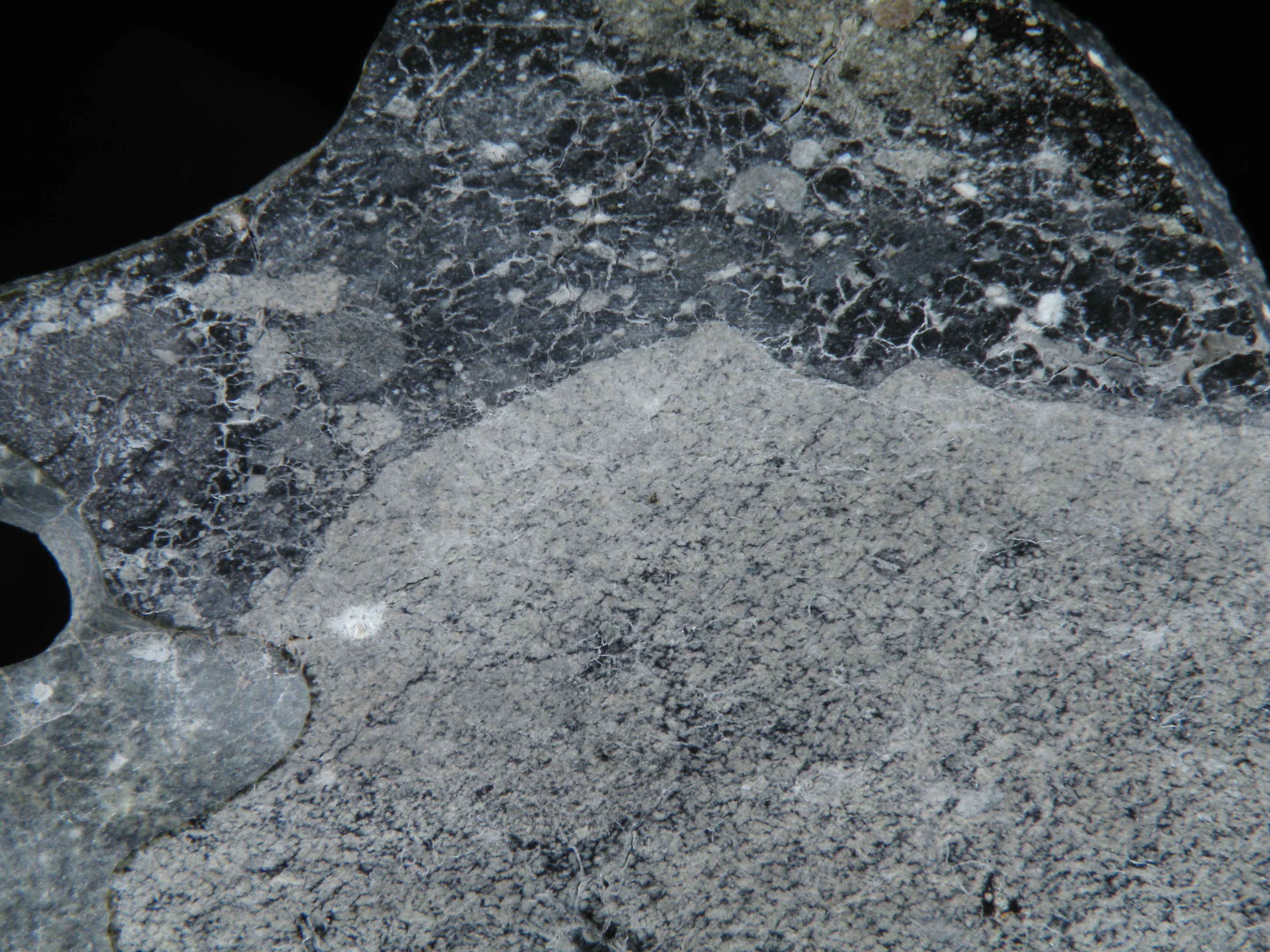
The wide range of chemically distinct achondrites tell us that many of these rocks formed in a variety of different environments, on different asteroids. Their ages and variations help us to fill in the evolving history of our solar system, from chondrules that somehow formed in the solar nebula, up until bigger planetesimals solidified and started bowling around the solar system.
Most iron meteorites also come from differentiated bodies, and they represent the more dense melt portions of thoroughly melted and recrystallized chondritic material. In other words, when you melt a big enough chondritic body (~10-20% Fe), you get an iron core. Smash a few of these huge bodies together, and you can actually expose some of that metal. Research into irons has shown us a great deal about the early planetismals in our solar system, and nuances of their chemistry have helped to pinpoint relationships between different chondrite groups and their respective melted counterparts. If you’d like to know more about iron meteorites, check out this page.
Stony-irons are a mixed-up group. Achondrites are crustal and mantle material from planetesimals, and irons are from similar bodies’ cores. Stony-irons are thought to represent the deep mantle to upper-core material from these same planetesimals. I found two great images from an unknown book that show what was going on quite clearly, but they were sourced from this page and didn’t have any associated copyright information.
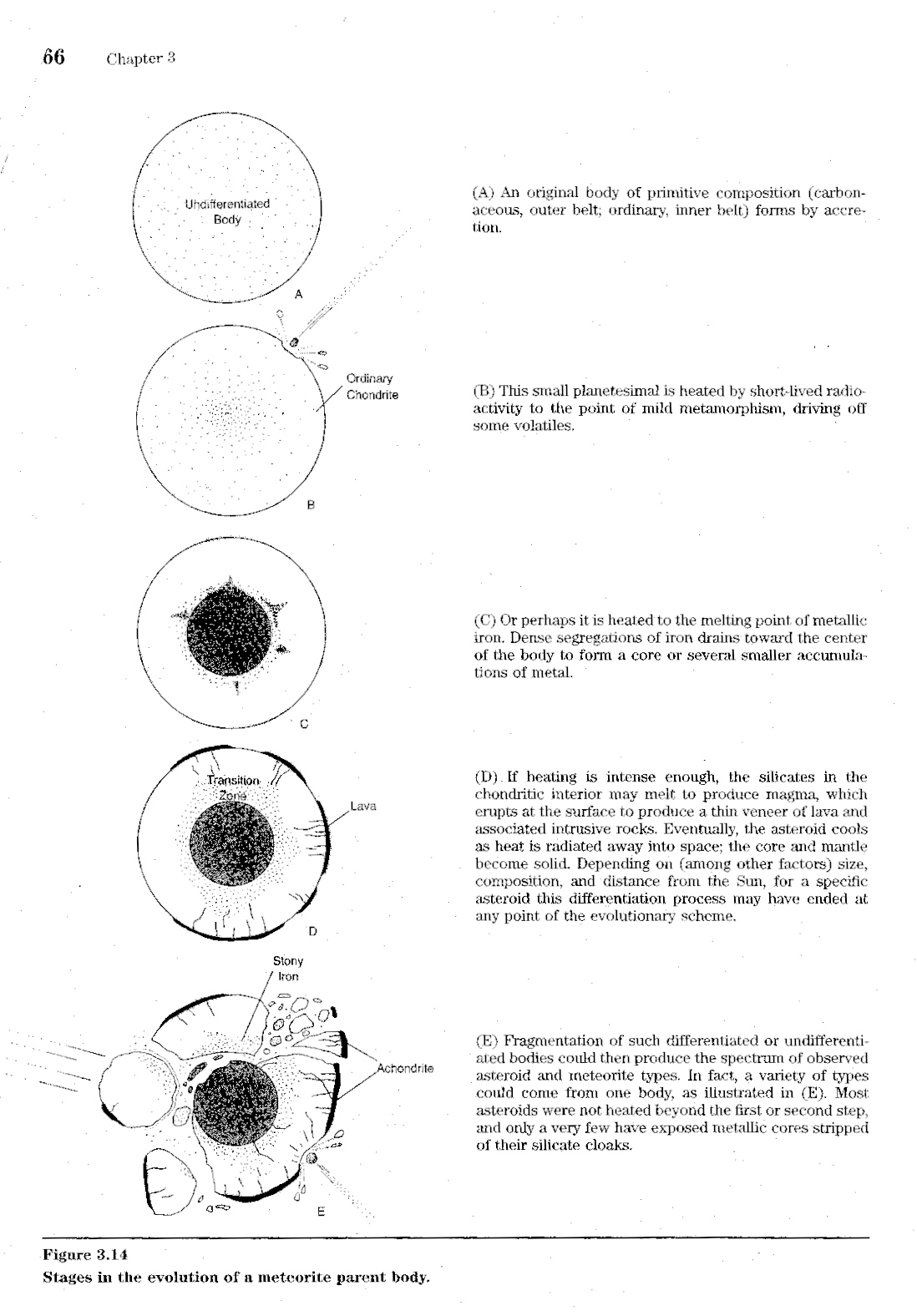
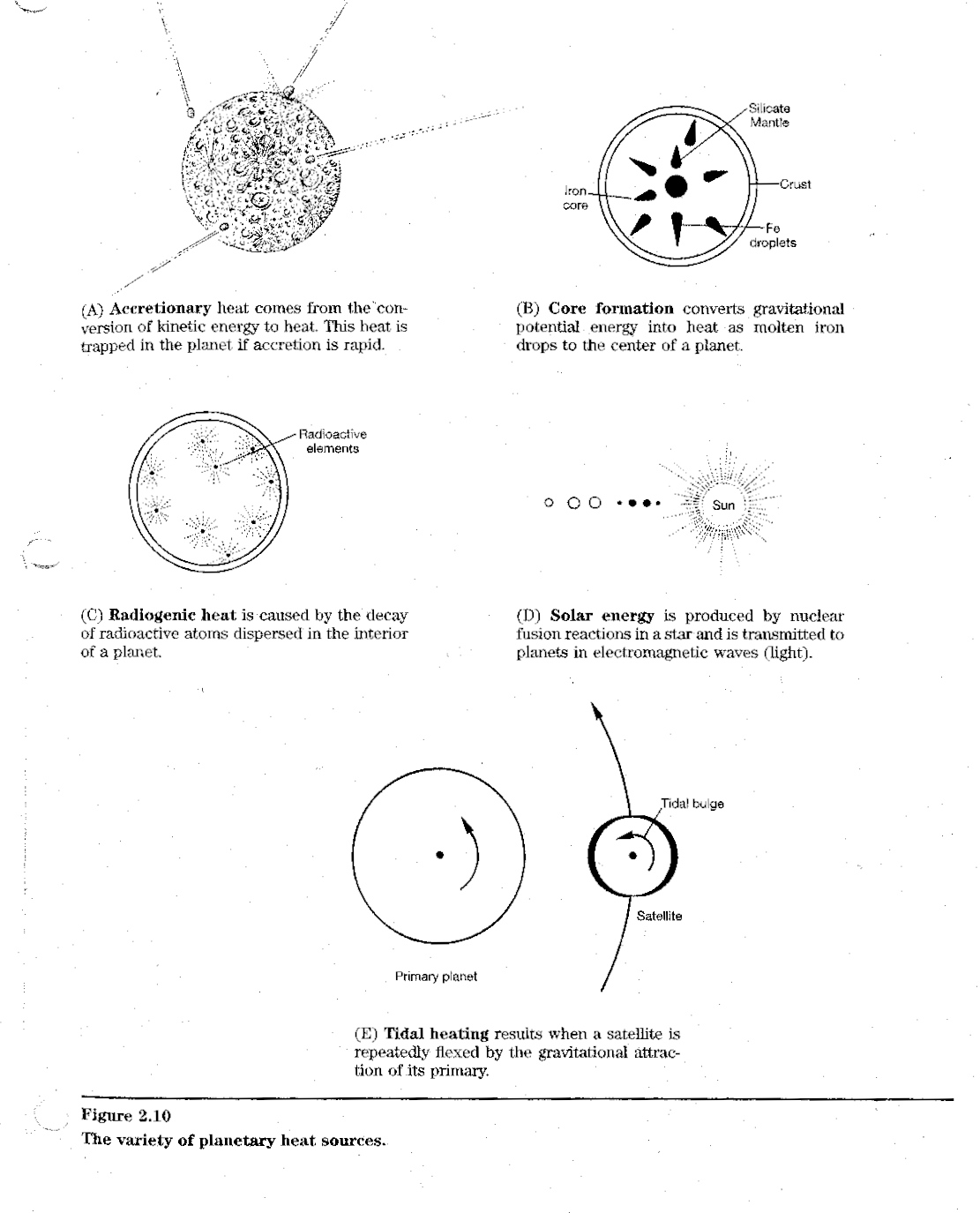
A thorough understanding of all of these rocks would help us understand what early conditions might result in a habitable body within a given solar system, to say nothing of expanding our ‘big picture’ knowledge of the universe. And it’s important to remember that our long-term survival as a species will depend upon a thorough understanding of planets and stellar systems. You don’t want to travel tens or hundreds of light years, only to find a star surrounded by an inhospitable planetary nebula. Or a solar system in which heavy elements are depleted or absent, etc. Our solar system seems to be somewhat atypical when we look around at nearby stars, and understanding what makes it special might be the key to the successful future of humanity.
I’ll be adding more links to relevant/important accessible papers in the future: please check back!