What are CAIs?
CAIs. Calcium aluminum inclusions. What’s so special about them and why do people who study meteorites talk about them so much?
Well, many people talk about chondrites being “four and a half billion years old.” And you wouldn’t be wrong if you said that. But, as is true with almost everything, when you look more closely, things get a little more interesting.
We don’t know exactly how chondrules and CAIs formed, but the abundances of isotopes in them tell us that they didn’t form in quite the same ways. CAIs more often show large variations in ratios of particular isotopes.
Crash course – stable isotope geochemistry
We observe isotope fractionation on Earth; it generally relies upon one simple principle: lighter atoms are easier to ‘move’ than heavier atoms. The classic example: rain. Water is H2O. Two hydrogen atoms and one oxygen atom. But not all oxygen is the same.
What does that mean?
All oxygen atoms have 8 protons. That’s what determines how many electrons they have, which determines how they can interact with other atoms and molecules. That is what makes them act like oxygen.
The top left number is the number of protons plus the number of neutrons in each atom.
So, in addition to those 8 protons, oxygen-16 has 8 neutrons in its nucleus, oxygen-17 has 9 neutrons, and oxygen-18 has 10 neutrons (it’s just addition). Neutrons add mass to atoms, but they aren’t charged particles, so they don’t change the structure of atoms’ electron shells. In other words, they add mass, but don’t change how atoms interact with other atoms in a large way. You can have “light” oxygen and “heavy” oxygen, but it’s still oxygen.
Let’s pay closer attention to three numbers in the above image: 15.9949, 16.9991, and 17.9991. Without getting too bogged down in the details. If you had 15.9949 pounds of 16O, the same number of atoms of 17O would weigh 16.9991 pounds — those 17O atoms would have an extra pound of neutrons in them. The same number of 18O atoms would weigh ~another extra pound.
Why is this important? If you think about different liquids — things like alcohol, water, engine coolant, or anything else — they all have different boiling points. Water boils at 212°F, engine coolant won’t boil until it gets really hot (up to ~356°F or so), and alcohol boils at just ~173°F. These differences in boiling point can be due to a few factors, it usually takes more energy to separate heavier molecules compared to lighter ones. Now, 16O is too close to 18O to have a measurably different boiling point, but when water evaporates, it is a little easier for the water with 16O to evaporate, because those atoms are a little lighter.
Think back to rain. When water evaporates, it’s a little easier for water molecules with those lighter atoms to take off. And when rain starts to fall, the water with heavier atoms condenses out a little more quickly: we observe this directly:
What’s going on here? Look at those ∂18O values. What do they mean? Well, we use the ratio of oxygen isotopes (16, 17, and 18) in global ocean water as a standard, so the value of ocean water is 0%0 for all three isotopes, by definition. You can see that in the lower left corner.
The water vapor in clouds over the ocean is a little light, because heavier water wanted to stay behind, as a liquid. It has less 18O in it than ocean water, by 12 parts per million (denoted by %0). You can see that in the top left corner of the image, where ∂18O = -12%0.
Even though the clouds are “light,” the first rain to fall is relatively enriched in 18O, because the heavy water wants to condense and drop out as a liquid. The resulting rain still has less 18O than ocean water, because the clouds had so little to begin with, but that rain is much more enriched in heavy water than the vapor that’s left behind. This keeps taking heavy oxygen out of the clouds, until they have a ∂18O value of -17%0 by the time they get inland.
Think about it this way: the heavy water likes condensing more than light water, so as the clouds drop more and more rain, the clouds lose heavy water and the clouds get “lighter.”
We see this in real life:
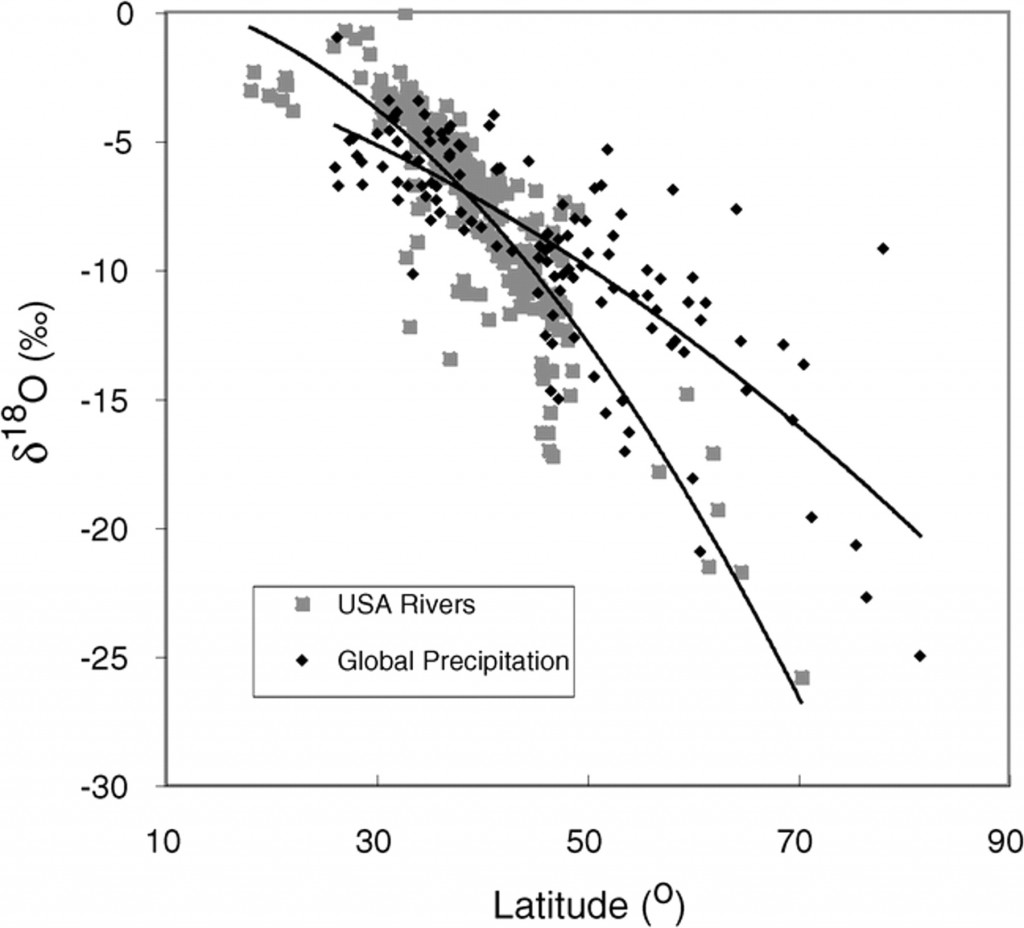
These kinds of reactions don’t just happen with water, though. They’re happening all around you, all the time. Plants concentrate light carbon isotopes because it’s easier for them to move lighter atoms around. Even your body is in on it: the nitrogen isotopic signature of herbivores is lighter than that of the plants they eat. Carnivores have even lighter nitrogen on average than herbivores. A person who eats meat will have a lighter nitrogen isotopic signature than a vegetarian. This is because lighter nitrogen isotopes are easier to move around in metabolic processes, so the effect is compounded with each trophic level. It’s the same principle as with O and C.
So…what about CAIs?
If you took a bunch of really hot gas with the composition of the Sun and you started to cool it, aluminum and calcium would be two of the first elements to condense — and then the first elements to solidify after things cooled down a bit more. We’ve dated CAIs; we know that they’re some of the earliest solid objects to condense in the solar system, at ~4.567 billion years old. So, the formation models and radiogenic dates support each other. That’s good.
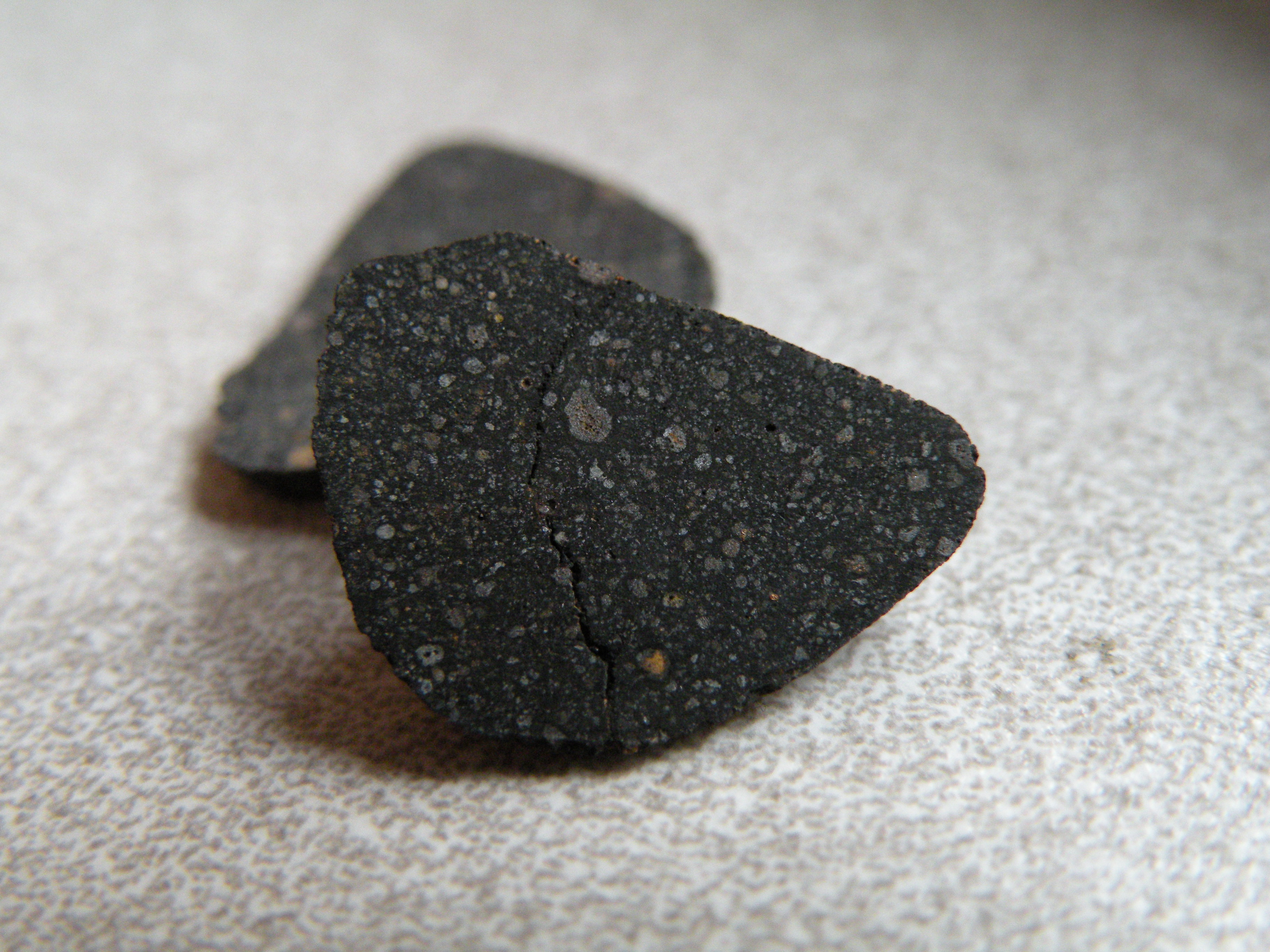
But why is this important? Well, if CAIs were some of the first solids to condense, they might preserve some evidence of what was going on in the solar nebula from…before most other things had formed.
And we see some interesting stuff in CAIs: some of them are seriously enriched in heavy isotopes, suggesting that they were exposed to heat capable of evaporating some of their (lighter) calcium and aluminum atoms. It’s just like we see on Earth, with water….but it was happening in space, over 4.5 billion years ago. But it’s not all so simple; some CAIs are messy. Some of the isotopic ratios we see suggest that these little globs of rock were exposed to widely fluctuating temperatures – they were evaporating at ~2,000+ °C, and then condensing — perhaps repeatedly. It’s difficult to tell how many heating events there might have been, but ongoing work on chondrules suggests that these events were likely repetitive or cyclical in nature.
So, while most CAIs have pretty simple isotopic signatures suggesting that they cooled right out of a gas, we know that the early Solar System wasn’t such a simple place everywhere. In places, at least, these little bits of rock formed and then were repeatedly evaporated and re-condensed. And there was definitely sorting going on; chemically different meteorites have different amounts of CAIs, with different average sizes, and slightly different compositions and textures.
In short, CAIs are some of the earliest solids we have examples of, and they have preserved at least a partial record of the earliest days of our Solar System. But, rocks are messy, there are a lot of isotopes, many of them are hard to measure precisely, and it’s hard to sort out exactly what they’re all telling us — that’s where research is ongoing.
This is why CAIs are cool.